Bea Baharier - From the San Rafael Swell to Mars
- Centa Conf 2020
- Aug 3, 2020
- 2 min read
Updated: Sep 14, 2020
Bea. Baharier *1, Julia Semprich 1, Karen Olsson-Francis1, Susanne. P. Schwenzer 1
1 AstrobiologyOU, School of Environment, Earth & Ecosystem Sciences, The Open University, Milton Keynes, MK7 6AA UK; Bea.baharier@open.ac.uk*1
The potential detection of present or past habitats beyond Earth is conducted by researching the geochemical and physical parameters. On Mars, remote sensing and ground-based rover missions acquire this data, which then is modelled and ground-truthed by analogue habitats on Earth. The geological history of Mars exhibits an early hospitable surface with an active hydrosphere, and a later hostile environment as Mars lost most of its atmosphere, magnetic field, surface water and was possibly dominated by sulphuric acid (Halevy et al., 2007; McLennan and Grotzinger, 2008). As Mars became less habitable, life started to appear on Earth (~3.8 Ga; Dodd et al., 2017). If microbes lived on the surface of Mars, they could have sheltered in isolated hydrothermal pools, within evaporites or in the subsurface (Westall et al., 2013; Michalski et al., 2013). This suggests that potential life on Mars may have evolved similarly to that of early Earth, merely earlier. Therefore, researching sulphur-rich hydrothermal habitats from Earth is key to finding putative life signs from the past or present on Mars. This study investigates the interaction between sulphate-rich sediment and magmatic intrusions, from the San Rafael Swell, Utah, USA, which may have created a sulphur-rich hydrothermal habitat. This is conducted by a field investigation and geochemical analysis. These results will then be used to model the water-rock interaction, and, finally, fluid habitability potential will be tested using microbial growth experiments. The implications of this search can assist in the search for habitable environments in NASA’s Mars 2020rover mission, planned to land at Jezero Crater and other future missions (Ehlmann and Mustard, 2012).
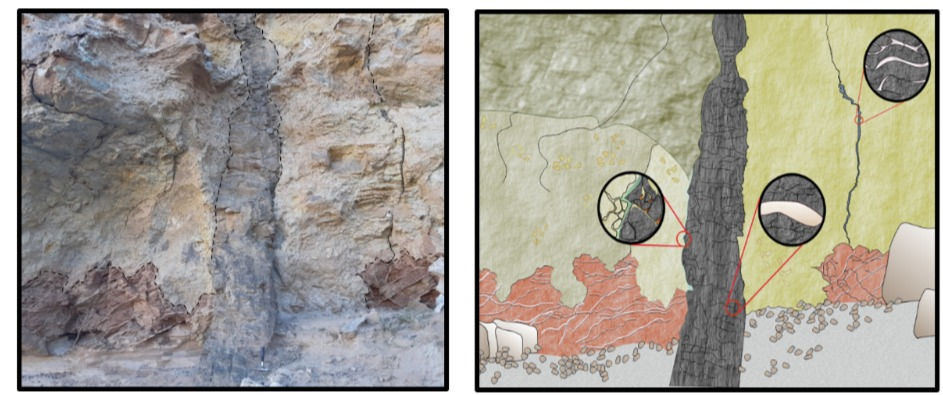
Fig 1. Left image of Awesome dyke and Baby dyke (to the right) intruded into the Jurassic sulphate-rich sediment San Raphael Swell, Utah, USA. The right image is a sketch of the same localitywith enlarged sketches from the left of (1) direct alteration zone (2) Sulphate vein within Awesome dyke and (3) Sulphate vein within Baby dyke.
References
Dodd, M.S., Papineau, D., Grenne, T., Slack, J.F., Rittner, M., Pirajno, F., O’Neil, J., Little, C.T.S., 2017. Evidence for early life in Earth’s oldest hydrothermal vent precipitates. Nature 543, 60–64. https://doi.org/10.1038/nature21377
Ehlmann, B.L., Mustard, J.F., 2012. An in-situ record of major environmental transitions on early Mars at Northeast Syrtis Major. Geophys. Res. Lett. 39, n/a-n/a. https://doi.org/10.1029/2012gl051594
Halevy, I., Zuber, M.T., Schrag, D.P., 2007. A Sulfur Dioxide Climate Feedback on Early Mars. Science 318, 1903–1907. https://doi.org/10.1126/science.1147039
Michalski, J.R., Cuadros, J., Niles, P.B., Parnell, J., Deanne Rogers, A., Wright, S.P., 2013. Groundwater activity on Mars and implications for a deep biosphere. Nature Geosci 6, 133–138. https://doi.org/10.1038/ngeo1706
McLennan, S. M., and & Grotzinger, J. P., (2008,) in J. F. Bell (ed.) The Martian Surface: 1336 Composition, Mineralogy and Physical Properties. Cambridge, 541-577, ISBN-13 978-0-1337 511-41372-8
Westall, F., Loizeau, D., Foucher, F., Bost, N., Betrand, M., Vago, J., Kminek, G., 2013. Habitability on Mars from a Microbial Point of View. Astrobiology 13, 887–897. https://doi.org/10.1089/ast.2013.1000
Comments